Olfactory neuronal precursors as a model to analyze the effects of melatonin in Alzheimer's disease.
Effects of melatonin in Alzheimer's disease
Abstract
Alzheimer's disease (AD) is a multifactorial disorder of great importance affecting millions globally and its prevalence will triple in the following decades. Therefore, analysis and identification of substances which can effectively reduce the pathological process of this disease in different study models are crucial. Melatonin works as a multitasking substance and some of its activities could be used to target the neurodegenerative process of AD. These include, but not limited to, its potent antioxidant activity, regulation of sleep-wake rhythms (important for the consolidation of memory and cognition) and its action as a neurotrophic growth factor that promotes differentiation and neuronal proliferation. To evaluate the effects of melatonin at cellular level in AD, it is essential to have a study model that reflects the pathological process occurring in the CNS. In this, review we summarize the potential use of olfactory neuronal precursors derived from olfactory neuroepithelium directly obtained from patients for such purposes.
References
2. Liu C, Fukuhara C, Wessel JH,. Iuvone PM, Tosini G (2004) Localization of Aa-nat mRNA in the rat retina by fluorescence in situ hybridization and laser capture microdissection. Cell Tissue Res. 315: 197–201.https://doi.org/10.1007/s00441-003-0822-1.
3. Conti A, Conconi S, Hertens E, Skwarlo-Sonta K, Markowska M, Maestroni JM (2000) Evidence for melatonin synthesis in mouse and human bone marrow cells. J. Pineal Res. 28: 193–202. https://doi.org/10.1034/j.1600-079x.2000.280401.x.
4. Tan DX, Manchester LC, Reiter RJ, Qi WB, Zhang M, Weintraub S T, Cabrera J, Sainz RM, Mayo JC (1999) Identification of highly elevated levels of melatonin in bone marrow: its origin and significance. Biochimica et Biophysica Acta (BBA) - General Subjects 1472: 206–214. https://doi.org/10.1016/s0304-4165(99)00125-7.
5. Slominski A, Pisarchik A, Zbytek B, Tobin DJ, Kauser S, Wortsman J (2003) Functional activity of serotoninergic and melatoninergic systems expressed in the skin. J. Cell Physiol. 196: 144–153. https://doi.org/10.1002/jcp.10287.
6. Stefulj J, Hörtner M, Ghosh M, Schauenstein K, Rinner I, Wölfler A, Semmler J, Liebmann PM (2001) Gene expression of the key enzymes of melatonin synthesis in extrapineal tissues of the rat. J. Pineal Res. 30: 243–247. https://doi.org/10.1034/j.1600-079x.2001.300408.x.
7. Claustrat B, Leston J (2015) Melatonin: Physiological effects in humans. Neurochirurgie 61: 77–84. https://doi.org/10.1016/j.neuchi.2015.03.002.
8. Cardinali DP (1981) Melatonin. A mammalian pineal hormone. Endocr. Rev. 2: 327–346. https://doi.org/10.1210/edrv-2-3-327.
9. Reiter RJ (1986) Normal patterns of melatonin levels in the pineal gland and body fluids of humans and experimental animals. J. Neural Transm. Suppl. 21: 35–54.
10. Reiter RJ (1993) The melatonin rhythm: both a clock and a calendar. Experientia 49: 654–664. https://doi.org/10.1007/BF01923947.
11. Miranda-Riestra A, Estrada-Reyes R, Torres-Sanchez ED, Carreño-García S, Ortiz GG, Benítez-King G (2022) Melatonin: A Neurotrophic Factor? Molecules 27: 7742. https://doi.org/10.3390/molecules27227742.
12. Winblad B, et al., (2016) Defeating Alzheimer’s disease and other dementias: a priority for European science and society. Lancet Neurol. 15: 455–532. https://doi.org/10.1016/S1474-4422(16)00062-4.
13. Gauthier S, Webster C, Servaes S, Morais JA, Rosa-Neto P (2022) World Alzheimer Report 2022: Life after diagnosis: Navigating treatment, care and support. Alzheimer’s Disease International. https://www.alzint.org/u/World-Alzheimer-Report-2022.pdf p.4.
14. Rajan KB, Weuve J, Barnes LL, McAninch EA, Wilson RS, Evans DA (2021) Population estimate of people with clinical Alzheimer’s disease and mild cognitive impairment in the United States (2020–2060). Alzheimer’s Dement. 17: 1966–1975. https://doi.org/10.1002/alz.12362.
15. Breijyeh Z, Karaman R (2020) Comprehensive Review on Alzheimer’s Disease: Causes and Treatment. Molecules 25: 5789. https://doi.org/10.3390/molecules25245789.
16. Serrano-Pozo A, Frosch MP, Masliah E, Hyman BT (2011) Neuropathological Alterations in Alzheimer Disease. Cold Spring Harb. Perspect. Med. 1: a006189–a006189. https://doi.org/10.1101/cshperspect.a006189.
17. Kent BA, Mistlberger RE (2017) Sleep and hippocampal neurogenesis: Implications for Alzheimer’s disease. Front. Neuroendocrinol. 45: 35–52. https://doi.org/10.1016/j.yfrne.2017.02.004.
18. Lanza G, DelRosso LM, Ferri R (2022) Sleep and homeostatic control of plasticity. Handb. Clin. Neurol. 184: 53–72. https://doi.org/10.1016/B978-0-12-819410-2.00004-7.
19. Maquet P (2001) The Role of Sleep in Learning and Memory. Science 294: 1048–1052. https://doi.org/10.1126/science.1062856.
20. Miyata S, et al., (2013) Poor sleep quality impairs cognitive performance in older adults. J. Sleep Res. 22: 535–541. https://doi.org/10.1111/jsr.12054.
21. Ma XQ, Jiang CQ, Xu L, Zhang WS, Zhu F, Jin YL, Thomas GN, Lam TH (2019) Sleep quality and cognitive impairment in older Chinese: Guangzhou Biobank Cohort Study. Age Ageing. 49: 119–124. https://doi.org/10.1093/ageing/afz120.
22. Guarnieri B, et al., (2012) Prevalence of sleep disturbances in mild cognitive impairment and dementing disorders: A multicenter italian clinical cross-sectional study on 431 patients. Dement. Geriatr. Cogn. Disord. 33: 50–58. https://doi.org/10.1159/000335363.
23. Moran M, et al., (2005) Sleep disturbance in mild to moderate Alzheimer’s disease. Sleep Med. 6: 347–352. https://doi.org/10.1016/j.sleep.2004.12.005.
24. Branger P, et al., (2016) Relationships between sleep quality and brain volume, metabolism, and amyloid deposition in late adulthood. Neurobiol. Aging. 41: 107–114. https://doi.org/10.1016/j.neurobiolaging.2016.02.009.
25. Brown BM, et al., (2016) The relationship between sleep quality and brain amyloid burden. Sleep. 39: 1063–1068. https://doi.org/10.5665/sleep.5756.
26. Mishima K, Tozawa T, Satoh K, Matsumoto Y, Hishikawa Y, Okawa M (1999) Melatonin secretion rhythm disorders in patients with senile dementia of Alzheimer’s type with disturbed sleep–waking. Biol. Psychiatry. 45: 417–421. https://doi.org/10.1016/s0006-3223(97)00510-6.
27. Tohgi H, et al., (1992) Concentrations of serotonin and its related substances in the cerebrospinal fluid in patients with Alzheimer type dementia. Neurosci. Lett. 141: 9–12. https://doi.org/10.1016/0304-3940(92)90322-x.
28. Brunner P, Sözer-Topcular N, Jockers R, Ravid R, Angeloni D, Fraschini F, Eckert A, Müller-Spahn F, Savaskan E (2006) Pineal and cortical melatonin receptors MT1 and MT2 are decreased in Alzheimer’s disease. Eur. J. Histochem. 50: 311–6.
29. Wade AG., et al., (2014) Add-on prolonged-release melatonin for cognitive function and sleep in mild to moderate Alzheimer’s disease: A 6-month, randomized, placebo-controlled, multicenter trial. Clin. Interv. Agin. 9: 947–961. https://doi.org/10.2147/CIA.S65625.
30. Gehrman PR, et al., (2009) Melatonin fails to improve sleep or agitation in double-blind randomized placebo-controlled trial of institutionalized patients with Alzheimer disease. The Am. J. Geriatr. Psychiatry. 17: 166–169. https://doi.org/10.1097/JGP.0b013e318187de18.
31. Xu L, Yu H, Sun H, Hu B, Geng Y (2020) Dietary melatonin therapy alleviates the lamina cribrosa damages in patients with mild cognitive impairments: A double-blinded, randomized controlled study. Medical Science Monitor. 26: e923232. https://doi.org/10.12659/MSM.923232
32. Domínguez-Alonso A, Ramírez-Rodríguez G, Benítez-King G (2012) Melatonin increases dendritogenesis in the hilus of hippocampal organotypic cultures. J. Pineal Res. 52: 427–436. https://doi.org/10.1111/j.1600-079X.2011.00957.x.
33. Jones DP (2006) Redefining Oxidative Stress. Antioxid. Redox. Signal. 8: 1865–1879. https://doi.org/10.1089/ars.2006.8.1865.
34. Butterfield DA, Halliwell B (2019) Oxidative stress, dysfunctional glucose metabolism and Alzheimer disease. Nat. Rev. Neurosci. 20: 148–160. https://doi.org/10.1038/s41583-019-0132-6.
35. Ionescu-Tucker A, Cotman CW (2021) Emerging roles of oxidative stress in brain aging and Alzheimer’s disease. Neurobiol. Aging 107: 86–95. https://doi.org/10.1016/j.neurobiolaging.2021.07.014.
36. Zhao Y, Zhao B (2013) Oxidative stress and the pathogenesis of Alzheimer’s disease. Oxid. Med. Cell Longev. 2013: 1–10. https://doi.org/10.1155/2013/316523.
37. Chen Z, Zhong C, (2014) Oxidative Stress in Alzheimer’s Disease. Neurosci. Bull. 30: 271–281. https://doi.org/10.1007/s12264-013-1423-y.
38. Reiter RJ, et al., (2016) Melatonin as an antioxidant: under promises but over delivers. J. Pineal Res. 61: 253–278. https://doi.org/10.1111/jpi.12360.
39. Tan DX, Manchester LC, Terron MP, Flores LJ, Reiter RJ (2007) One molecule, many derivatives: A never-ending interaction of melatonin with reactive oxygen and nitrogen species? J. Pineal Res. 42: 28–42. https://doi.org/10.1111/j.1600-079X.2006.00407.x.
40. Masilamoni JG, et al., (2008) The neuroprotective role of melatonin against amyloid β peptide injected mice. Free Radic. Res. 42: 661–673. https://doi.org/10.1080/10715760802277388.
41. Solís-Chagoyán H, Domínguez-Alonso A, Valdés-Tovar M, Argueta J, Sánchez-Florentino ZA, Calixto E, Benítez-King G (2020) Melatonin rescues the dendrite collapse induced by the pro-oxidant toxin okadaic acid in organotypic cultures of rat hilar hippocampus. Molecules 25: 5508.
42. Deng Y, Xu G, Duan P, Zhang Q, Wang J (2005) Effects of melatonin on wortmannin-induced tau hyperphosphorylation. Acta Pharmacol. Sin. 26: 519–526. https://doi.org/10.1111/j.1745-7254.2005.00102.x.
43. Altman J (1962) Are new neurons formed in the brains of adult mammals? Science 135: 1127–1128. https://doi.org/10.1126/science.135.3509.1127.
44. Altman J, Das GD (1965) Autoradiographic and histological evidence of postnatal hippocampal neurogenesis in rats. J. Comp. Neurol. 124: 319–335. https://doi.org/10.1002/cne.901240303.
45. Abrous DN, Koehl M, Le Moal M (2005) Adult neurogenesis: from precursors to network and physiology. Physiol. Rev. 85: 523–569. https://doi.org/10.1152/physrev.00055.2003.
46. Taupin P, Gage FH (2002) Adult neurogenesis and neural stem cells of the central nervous system in mammals. J. Neurosci. Res. 69: 745–749. https://doi.org/10.1002/jnr.10378.
47. Mathews KJ, et al., (2017) Evidence for reduced neurogenesis in the aging human hippocampus despite stable stem cell markers. Aging Cell. 16: 1195–1199. https://doi.org/10.1111/acel.12641.
48. von Bohlen und Halbach O (2011) Immunohistological markers for proliferative events, gliogenesis, and neurogenesis within the adult hippocampus. Cell Tissue Res. 345: 1–19. https://doi.org/10.1007/s00441-011-1196-4.
49. Moriya T, Horie N, Mitome M, Shinohara K (2007) Melatonin influences the proliferative and differentiative activity of neural stem cells. J. Pineal Res. 42: 411–418. https://doi.org/10.1111/j.1600-079X.2007.00435.x.
50. Fu J, et al., (2011) Melatonin promotes proliferation and differentiation of neural stem cells subjected to hypoxia in vitro. J. Pineal Res. 51: 104–112. https://doi.org/10.1111/j.1600-079X.2011.00867.x.
51. Sotthibundhu A, Phansuwan-Pujito P, Govitrapong P (2010) Melatonin increases proliferation of cultured neural stem cells obtained from adult mouse subventricular zone. J. Pineal Res. 49: 291–300. https://doi.org/10.1111/j.1600-079X.2010.00794.x.
52. Song J, Kang SM, Lee KM, Lee JE (2015) The Protective Effect of Melatonin on Neural Stem Cell against LPS-Induced Inflammation. Biomed. Res. Int. 2015: 1–13. https://doi.org/10.1155/2015/854359.
53. Ramirez-Rodriguez G, Ortíz-López L, Domínguez-Alonso A, Benítez-King G, Kempermann G (2011) Chronic treatment with melatonin stimulates dendrite maturation and complexity in adult hippocampal neurogenesis of mice. J. Pineal, Res. 50: 29–37. https://doi.org/10.1111/j.1600-079X.2010.00802.x.
54. Moreno-Jiménez EP, et al., (2019) Adult hippocampal neurogenesis is abundant in neurologically healthy subjects and drops sharply in patients with Alzheimer’s disease. Nat. Med. 25: 554–560. https://doi.org/10.1038/s41591-019-0375-9.
55. Tobin MK, et al., (2019) Human hippocampal neurogenesis persists in aged adults and Alzheimer’s disease patients. Cell Stem Cell. 24: 974–982. https://doi.org/10.1016/j.stem.2019.05.003.
56. Jin K, et al., (2004) Increased hippocampal neurogenesis in Alzheimer’s disease. Proceedings of the National Academy of Sciences. 101: 343–347. https://doi.org/10.1073/pnas.2634794100.
57. Chandra A, Dervenoulas G, Politis M (2019) Magnetic resonance imaging in Alzheimer’s disease and mild cognitive impairment. J. Neurol. 206: 1293–1302. https://doi.org/10.1007/s00415-018-9016-3.
58. 2023 Alzheimer's disease facts and figures. (2023). Alzheimer's Dement. 19: 1598–1695. https://doi.org/10.1002/alz.13016
59. González-Burgos I, Letechipía-Vallejo G, López-Loeza E, Moralí G, Cervantes M (2007) Long-term study of dendritic spines from hippocampal CA1 pyramidal cells, after neuroprotective melatonin treatment following global cerebral ischemia in rats. Neurosci. Lett. 423: 162–166. https://doi.org/10.1016/j.neulet.2007.06.050.
60. Alghamdi BS, AboTaleb HA (2020) Melatonin improves memory defects in a mouse model of multiple sclerosis by up-regulating cAMP-response element-binding protein and synapse-associated proteins in the prefrontal cortex. J. Integr. Neurosci. 19: 229. https://doi.org/10.31083/j.jin.2020.02.32.
61. Permpoonputtana K, Tangweerasing P, Mukda S, Boontem P, Nopparat C, Govitrapong P (2018) Long-term administration of melatonin attenuates neuroinflammation in the aged mouse brain. EXCLI J. 17: 634–646. https://doi.org/10.17179/excli2017-654.
62. Labban S, Alghamdi BS, Alshehri FS, Kurdi M (2021) Effects of melatonin and resveratrol on recognition memory and passive avoidance performance in a mouse model of Alzheimer’s disease. Behav. Brain Res. 402: 113100. https://doi.org/10.1016/j.bbr.2020.113100.
63. O’Neal-Moffitt G, Delic V, Bradshaw PC, Olcese J (2015) Prophylactic melatonin significantly reduces Alzheimer’s neuropathology and associated cognitive deficits independent of antioxidant pathways in AβPPswe/PS1 mice. Mol. Neurodegener. 10: 27. https://doi.org/10.1186/s13024-015-0027-6.
64. Cardinali DP, Vigo DE, Olivar N, Vidal MF, Furio AM, Brusco LI (2012) Application of melatonin in mild cognitive impairment. Am. J. Neurodegener. Dis. 1: 280–291.
65. Furio AM, Brusco LI, Cardinali DP (2007) Possible therapeutic value of melatonin in mild cognitive impairment: A retrospective study. J. Pineal. Res 43: 404–409. https://doi.org/10.1111/j.1600-079X.2007.00491.x.
66. Jafek BW, Murrow B, Michaels R, Restrepo D, Linschoten M (2002) Biopsies of human olfactory epithelium. Chem. Senses 27: 623–628. https://doi.org/10.1093/chemse/27.7.623.
67. Smith TD, Bhatnagar KP (2019) Anatomy of the olfactory system. Handb. Clin. Neurol.164: 17–28. https://doi.org/10.1016/B978-0-444-63855-7.00002-2.
68. Roisen FJ, Klueber KM, Lu CL, Hatcher LM, Dozier A, Shields CB, Maguire S (2001) Adult human olfactory stem cells. Brain Res. 890: 11–22. https://doi.org/10.1016/s0006-8993(00)03016-x.
69. Durante MA, et al., (2020) Single-cell analysis of olfactory neurogenesis and differentiation in adult humans. Nat. Neurosci. 23: 323–326. https://doi.org/10.1038/s41593-020-0587-9.
70. Evgrafov OV, et al., (2011) Olfactory neuroepithelium-derived neural progenitor cells as a model system for investigating the molecular mechanisms of neuropsychiatric disorders. Psychiatr. Genet. 21: 217–228. https://doi.org/10.1097/YPG.0b013e328341a2f0.
71. Zhang X, Klueber KM, Guo Z, Cai J, Lu C, Winstead WI, Qiu M, Roisen FJ (2006) Induction of neuronal differentiation of adult human olfactory neuroepithelial-derived progenitors. Brain Res. 1073–1074: 109–119. https://doi.org/10.1016/j.brainres.2005.12.059.
72. Borgmann-Winter KE, et al., (2009) Human olfactory epithelial cells generated in vitro express diverse neuronal characteristics. Neuroscience 158: 642–653. doi: 10.1016/j.neuroscience.2008.09.059. Epub 2008 Oct 17. PMID: 18996445; PMCID: PMC2951119.
73. Galván-Arrieta T, et al., (2017) The role of melatonin in the neurodevelopmental etiology of schizophrenia: A study in human olfactory neuronal precursors. J. Pineal. Res. 63. https://doi.org/10.1111/jpi.12421.
74. Klimmeck D, et al., (2008) Calcium-signaling networks in olfactory receptor neurons. Neuroscience 151: 901–912. https://doi.org/10.1016/j.neuroscience.2007.11.023.
75. Tajinda K, et al., (2010) Neuronal biomarkers from patients with mental illnesses: a novel method through nasal biopsy combined with laser-captured microdissection. Mol. Psychiatry. 15: 231–232. https://doi.org/10.1038/mp.2009.73.
76. Féron F, Perry C, McGrath JJ, Mackay-Sim A (1998) New Techniques for Biopsy and Culture of Human Olfactory Epithelial Neurons. Arch. Otolaryngol. Head Neck Surg. 124: 861–866. https://doi.org/10.1001/archotol.124.8.861.
77. Féron F, Perry C, Hirning MH, McGrath J, Mackay-Sim A (1999) Altered adhesion, proliferation and death in neural cultures from adults with schizophrenia. Schizophr. Res. 40: 211–218. https://doi.org/10.1016/s0920-9964(99)00055-9.
78. Benítez-King G, et al., (2011) A non-invasive method to isolate the neuronal linage from the nasal epithelium from schizophrenic and bipolar diseases. J. Neurosci. Methods. 201: 35–45. https://doi.org/10.1016/j.jneumeth.2011.07.009.
79. Horiuchi Y, Kondo MA, Okada K, Takayanagi Y, Tanaka T, Ho T, Varvaris M, Tajinda K, Hiyama H, Ni K, Colantuoni C, Schretlen D, Cascella NG, Pevsner J, Ishizuka K, Sawa A, (2016) Molecular signatures associated with cognitive deficits in schizophrenia: A study of biopsied olfactory neural epithelium. Transl. Psychiatry, 6: e915. https://doi.org/10.1038/tp.2016.154.
80. Idotta C, et al., (2019) Olfactory neuroepithelium alterations and cognitive correlates in schizophrenia. Eur. Psychiatry 61: 23–32. https://doi.org/10.1016/j.eurpsy.2019.06.004.
81. Wolozin B, Bacic M, Merrill MJ, Lesch KP, Chen C, Lebovics RS, Sunderland T (1992) Differential expression of carboxyl terminal derivatives of amyloid precursor protein among cell lines. J. Neurosci. Res. 33: 163–9. https://doi.org/10.1002/jnr.490330121.
82. Wolozin B, Sunderland T, Zheng BB, Resau J, Dufy B, Barker J, Swerdlow R, Coon H (1992) Continuous culture of neuronal cells from adult human olfactory epithelium. Journal of Molecular Neuroscience 3: 137–146. https://doi.org/10.1007/BF02919405.
83. Wolozin B, Lesch P, Lebovics R, Sunderland T (1993) Olfactory neuroblasts from Alzheimer donors: Studies on APP processing and cell regulation. Biol. Psychiatry. 34: 824–838. https://doi.org/10.1016/0006-3223(93)90051-E.
84. Johnson GS, et al., (1994) Protein alterations in olfactory neuroblasts from Alzheimer donors. Neurobiol. Aging. 15: 675–680. https://doi.org/10.1016/0197-4580(94)90048-5.
85. Arnold SE, et al., (2010) Olfactory epithelium amyloid-β and paired helical filament-tau pathology in Alzheimer disease. Ann. Neurol. 67: 462–469. https://doi.org/10.1002/ana.21910.
86. Godoy MDCL, et al., (2019) Is olfactory epithelium biopsy useful for confirming Alzheimer’s disease? Ann. Otol. Rhinol. Laryngol. 128: 184–192. https://doi.org/10.1177/0003489418814865.
87. Ayala-Grosso CA, et al., (2015) Amyloid-Aβ peptide in olfactory mucosa and mesenchymal stromal cells of mild cognitive impairment and Alzheimer’s disease patients. Brain Pathol. 25: 136–145. https://doi.org/10.1111/bpa.12169.
88. Riquelme A, et al., (2020) Potential use of exfoliated and cultured olfactory neuronal precursors for in vivo Alzheimer’s disease diagnosis: A pilot study. Cell Mol. Neurobiol. 40: 87–98. https://doi.org/10.1007/s10571-019-00718-.
89. Getchell ML, Shah DS, Buch SK, Davis DG, Getchell TV (2003) 3-Nitrotyrosine immunoreactivity in olfactory receptor neurons of patients with Alzheimer’s disease: implications for impaired odor sensitivity. Neurobiol. Aging. 24: 663–673. https://doi.org/10.1016/s0197-4580(02)00195-1.
90. Ogawa T, Ohira A, Amemiya T (1997) Manganese ad copper-zinc superoxide dismutases in the developing rat retina. Acta Histochem. 99: 1–12. https://doi.org/10.1016/s0065-1281(97)80002-5.
91. Ghanbari HA, et al., (2004) Oxidative damage in cultured human olfactory neurons from Alzheimer’s disease patients. Aging Cell 3: 41–44. https://doi.org/10.1111/j.1474-9728.2004.00083.x.
92. Nelson VM, Dancik CM, Pan W, Jiang ZG, Lebowitz MS, Ghanbari HA (2009) PAN-811 inhibits oxidative stress-induced cell death of human alzheimer’s disease-derived and age-matched olfactory neuroepithelial cells via suppression of intracellular reactive oxygen species. Journal of Alzheimer’s Dis. 17: 611–619. https://doi.org/10.3233/JAD-2009-1078.
93. Graziadei PPC, Karlan MS, Monti GA, Bernstein JJ (1980) Neurogenesis of sensory neurons in the primate olfactory system after section of the fila olfactoria. Brain Res. 186: 289–300. https://doi.org/10.1016/0006-8993(80)90976-2.
94. Calof AL, Mumm JS, Rim PC, Shou J (1998) The neuronal stem cell of the olfactory epithelium. J. Neurobiol. 36: 190–205. https://doi.org/10.1002/(sici)1097-4695(199808)36:2<190::aid-neu7>3.0.co;2-x.
95. Fatemi SH, Folsom TD (2009) The neurodevelopmental hypothesis of schizophrenia, revisited. Schizophr. Bull. 35: 528–548. https://doi.org/10.1093/schbul/sbn187.
96. McCurdy RD, et al., (2006) Cell cycle alterations in biopsied olfactory neuroepithelium in schizophrenia and bipolar I disorder using cell culture and gene expression analyses. Schizophr. Res. 82: 163–173. https://doi.org/10.1016/j.schres.2005.10.012.
97. Arnold SE, et al., (2001) Dysregulation of olfactory receptor neuron lineage in schizophrenia. Arch. Gen. Psychiatry. 58: 829–835. https://doi.org/10.1001/archpsyc.58.9.829.
98. Solís-Chagoyán H, et al., (2013) Microtubule organization and L-type voltage-activated calcium current in olfactory neuronal cells obtained from patients with schizophrenia and bipolar disorder. Schizophr. Res. 143: 384–389. https://doi.org/10.1016/j.schres.2012.11.035.
99. Brown AS, et al., (2014) Increased stability of microtubules in cultured olfactory neuroepithelial cells from individuals with schizophrenia. Prog.Neuro-psychopharmacol. Biol. Psychiatry 48: 252–258. https://doi.org/10.1016/j.pnpbp.2013.10.015.
100. Muñoz-Estrada J, Benítez-King G, Berlanga C, Meza I (2015) Altered subcellular distribution of the 75-kDa DISC1 isoform, cAMP accumulation, and decreased neuronal migration in schizophrenia and bipolar disorder: Implications for neurodevelopment. CNS. Neurosci. Ther. 21: 446–453. https://doi.org/10.1111/cns.12377.
101. Benitez-King G (2006) Melatonin as a cytoskeletal modulator: implications for cell physiology and disease. J. Pineal. Res. 40: 1–9. https://doi.org/10.1111/j.1600-079X.2005.00282.x.
102. Cercós MG, et al., (2017) Abnormally increased secretion in olfactory neuronal precursors from a case of schizophrenia is modulated by melatonin: A pilot study. Int. J. Mol. Sci. 18: 1439. https://doi.org/10.3390/ijms18071439.
103. Estrada-Reyes R, et al., (2022) Antidepressant low doses of ketamine and melatonin in combination produce additive neurogenesis in human olfactory neuronal precursors. Molecules 27: 5650. https://doi.org/10.3390/molecules27175650.
104. Gilliland CT, Sittampalam GS, Wang PY, Ryan PE (2017) The translational science training program at NIH: Introducing early career researchers to the science and operation of translation of basic research to medical interventions. Biochem. Mol. Biol.Educ. 45: 13–24. https://doi.org/10.1002/bmb.20978.
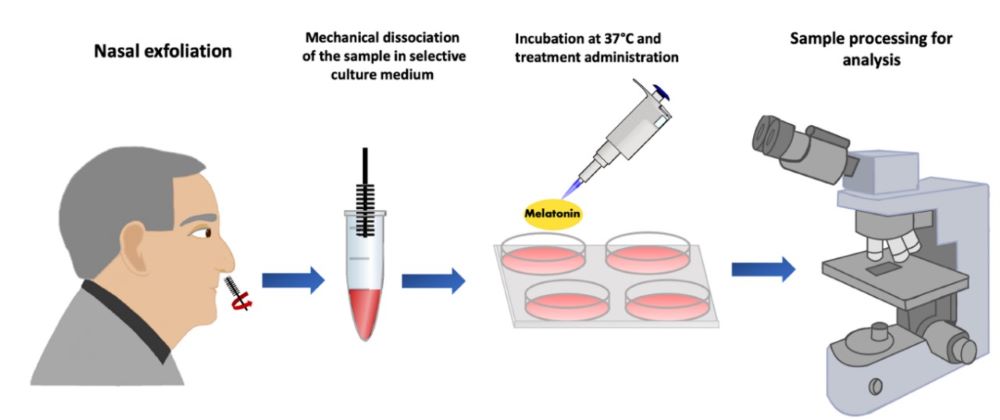

This work is licensed under a Creative Commons Attribution 4.0 International License.
For all articles published in Melatonin Res., copyright is retained by the authors. Articles are licensed under an open access Creative Commons CC BY 4.0 license, meaning that anyone may download and read the paper for free. In addition, the article may be reused and quoted provided that the original published version is cited. These conditions allow for maximum use and exposure of the work, while ensuring that the authors receive proper credit.
In exceptional circumstances articles may be licensed differently. If you have specific condition (such as one linked to funding) that does not allow this license, please mention this to the editorial office of the journal at submission. Exceptions will be granted at the discretion of the publisher.