Hyperbaric oxygen-assisted melatonin therapy protects the heart from acute ischemia-reperfusion injury
HBO and melatonin against IR
Abstract
In this study we have examined whether hyperbaric oxygen (HBO)-assisted melatonin (Mel) therapy effectively preserves the heart function against ischemia (40-min)-reperfusion (IR) injury. First, the in vitro study has been performed by use of cell culture. H9C2 cells were treated as groups: A (H9C2) (without any treatment), B (H9C2+IR), C (H9C2+IR+HBO), D [H9C2+IR+Melatonin (50 µM)] and E (H9C2+IR+Melatonin+HBO). The result showed that the protein expressions of oxidative-stress (NOX-1/NOX-2)/inflammatory (TNF-α/NF-κB)/apoptotic (mitochondrial-Bax/cleaved-caspase-3/cleaved-PARP) and cellular levels of DNA/mitochondrial-damaged (γ-H2AX/XRCC1-CD90+/cytosolic-cytochrome-C) biomarker were significantly increased in group B compared to control group A. These biomarkers were significantly reduced in group C, D and E compared to groups B with the significantly higher reduction in group E than that in groups C and D (p<0.001). In the in vivo study, adult-male SD rats (n=40) were equally divided into group 1 (sham-operated-control), group 2 (IR), group 3 (IR+HBO therapy at 1.5/24/48h after IR procedure), group 4 [IR+Mel (50mg/kg at 1.5h, followed by 20mg/kg and at days 1/2/3 after IR)] and group 5 (IR+HBO-Mel) and the heart was harvested at 72h after IR. The result showed that at 72h, the circulating levels of endothelial-progenitor-cells (c-kit-CD31+/CD31-sca-1+/KDR-CD34+/VE-Cadherin-CD34+) were lowest in group 2, highest in group 5 and followed by groups 3 > 4 >1. The significant differences were present between each two matched groups (p<0.0001). The protein expressions of angiogenic factors (SDF-1α/CXCR4/VEGF/HIF-α) were progressively increased from groups 1 to 5 with significant differences. The protein expressions of apoptosis (mitochondrial-Bax/cleaved-caspase-3/cleaved-PARP)/fibrosis (TGF-ß/Smad3)/oxidative-stress (NOX-1/NOX-2/oxidized protein)/inflammation (TNF-α/IL-1ß/MMP-9) and infarct/fibrotic areas were significantly increased in group 2 compared to the control group 1. All these parameters were significantly reduced in groups 3-5 compared to group 2 with significantly lowest level in group 5 among groups 3-5 (p < 0.01), whereas the left-ventricular-ejection-fraction exhibited an opposite pattern compared to the inflammatory factors. In conclusion, HBO-Mel therapy offered a synergic benefit for protecting the heart from IR injury.
References
2. Roth GA et al. (2017) Global, regional, and national burden of cardiovascular diseases for 10 causes, 1990 to 2015. J. Am. Coll Cardiol. 70 (1): 1-25.
3. Fischer Q et al. (2018) Optimal long-term antithrombotic treatment of patients with stable coronary artery disease and atrial fibrillation: "OLTAT registry". Int. J. Cardiol. 264: 64-69.
4. Gharacholou SM et al. (2018) Characteristics and long term outcomes of patients with acute coronary syndromes due to culprit left main coronary artery disease treated with percutaneous coronary intervention. Am. Heart J. 199: 156-162.
5. Medina-Inojosa JR et al. (2018) Association between adiposity and lean mass with long-term cardiovascular events in patients with coronary artery disease: No paradox. J. Am. Heart Assoc. 7 (10): e007505.
6. Kloner RA, Ellis SG, Lange R, Braunwald E (1983) Studies of experimental coronary artery reperfusion. Effects on infarct size, myocardial function, biochemistry, ultrastructure and microvascular damage. Circulation 68 (2 Pt 2): I8-15.
7. Ito H et al. (1996) Clinical implications of the 'no reflow' phenomenon. A predictor of complications and left ventricular remodeling in reperfused anterior wall myocardial infarction. Circulation 93 (2): 223-228.
8. Chen Y S et al. (2003) Analysis and results of prolonged resuscitation in cardiac arrest patients rescued by extracorporeal membrane oxygenation. J. Am. Coll. Cardiol. 41 (2): 197-203.
9. Rokos IC et al. (2006) Rationale for establishing regional ST-elevation myocardial infarction receiving center (SRC) networks. Am. Heart J. 152 (4): 661-667.
10. Rokos IC et al. (2009) Integration of pre-hospital electrocardiograms and ST-elevation myocardial infarction receiving center (SRC) networks: impact on Door-to-Balloon times across 10 independent regions. JACC Cardiovasc. Interv. 2 (4): 339-346.
11. Braunwald E, Kloner RA (1985) Myocardial reperfusion: a double-edged sword? J. Clin. Invest. 76 (5): 1713-1719.
12. De Scheerder I et al. (1985) Post-cardiac injury syndrome and an increased humoral immune response against the major contractile proteins (actin and myosin). Am. J. Cardiol. 56 (10): 631-633.
13. Frangogiannis NG (2008) The immune system and cardiac repair. Pharmacol. Res. 58 (2): 88-111.
14. Frangogiannis NG, Smith CW, Entman ML (2002) The inflammatory response in myocardial infarction. Cardiovasc. Res. 53 (1): 31-47.
15. Lambert JM, Lopez EF, Lindsey ML (2008) Macrophage roles following myocardial infarction. Int. J. Cardiol. 130 (2): 147-158.
16. Lange LG, Schreiner GF (1994) Immune mechanisms of cardiac disease. N. Engl. J. Med. 330 (16): 1129-1135.
17. Moens AL, Claeys MJ, Timmermans JP, Vrints CJ (2005) Myocardial ischemia/reperfusion-injury, a clinical view on a complex pathophysiological process. Int. J. Cardiol. 100 (2): 179-190.
18. Yellon DM, Hausenloy DJ (2007) Myocardial reperfusion injury. N. Engl. J. Med. 357 (11): 1121-1135.
19. Chang CL et al. (2015) Protective effect of melatonin-supported adipose-derived mesenchymal stem cells against small bowel ischemia-reperfusion injury in rat. J. Pineal Res. 59 (2): 206-220.
20. Chen HH et al. (2014) Additional benefit of combined therapy with melatonin and apoptotic adipose-derived mesenchymal stem cell against sepsis-induced kidney injury. J. Pineal Res. 57 (1): 16-32.
21. Manchester LC et al. (2015) Melatonin: an ancient molecule that makes oxygen metabolically tolerable. J. Pineal Res. 59 (4): 403-419.
22. Sun CK et al. (2015) Systemic combined melatonin-mitochondria treatment improves acute respiratory distress syndrome in the rat. J. Pineal Res. 58 (2): 137-150.
23. Yip HK et al. (2015) Combined melatonin and exendin-4 therapy preserves renal ultrastructural integrity after ischemia-reperfusion injury in the male rat. J. Pineal Res. 59 (4): 434-447.
24. Chen HH et al. (2016) Melatonin pretreatment enhances the therapeutic effects of exogenous mitochondria against hepatic ischemia-reperfusion injury in rats through suppression of mitochondrial permeability transition. J. Pineal Res. 61 (1): 52-68.
25. Sun CK et al. (2017) Melatonin treatment enhances therapeutic effects of exosomes against acute liver ischemia-reperfusion injury. Am. J. Transl. Res. 9 (4): 1543-1560.
26. Slovut DP, Sullivan TM (2008) Critical limb ischemia: medical and surgical management. Vasc. Med. 13 (3): 281-291.
27. Ko SF et al. (2020) Protective effect of combined therapy with hyperbaric oxygen and autologous adipose-derived mesenchymal stem cells on renal function in rodent after acute ischemia-reperfusion injury. Am. J. Transl. Res. 12 (7): 3272-3287.
28. Lin PY et al. (2018) Hyperbaric oxygen therapy enhanced circulating levels of endothelial progenitor cells and angiogenesis biomarkers, blood flow, in ischemic areas in patients with peripheral arterial occlusive disease. J. Clin. Med. 7 (12) :548.
29. Thom SR (2011) Hyperbaric oxygen: its mechanisms and efficacy. Plast. Reconstr. Surg. 127 Suppl 1: 131S-141S.
30. Lee FY et al. (2018) Combined therapy with SS31 and mitochondria mitigates myocardial ischemia-reperfusion injury in rats. Int. J. Mo.l Sci. 19 (9): 2782.
31. Hsu SL et al. (2019) Hyperbaric oxygen facilitates the effect of endothelial progenitor cell therapy on improving outcome of rat critical limb ischemia. Am. J. Transl. Res. 11 (4): 1948-1964.
32. Lin KC et al. (2019) Combined therapy with hyperbaric oxygen and melatonin effectively reduce brain infarct volume and preserve neurological function after acute ischemic infarct in rat. J. Neuropathol. Exp. Neurol. 78 (10): 949-960.
33. Chua S et al. (2014) Inhibition of dipeptidyl peptidase-IV enzyme activity protects against myocardial ischemia-reperfusion injury in rats. J. Transl. Med. 12: 357.
34. Yeh KH et al. (2012) Benefit of combined extracorporeal shock wave and bone marrow-derived endothelial progenitor cells in protection against critical limb ischemia in rats. Crit. Care Med. 40 (1): 169-177.
35. Lu HI et al. (2017) SS31 therapy effectively protects the heart against transverse aortic constriction-induced hypertrophic cardiomyopathy damage. Am. J. Transl. Res. 9 (12): 5220-5237.
36. Chen YC et al. (2020) Circulatory rejuvenated EPCs derived from PAOD patients treated by CD34(+) cells and hyperbaric oxygen therapy salvaged the nude mouse limb against critical ischemia. Int. J. Mol. Sci. 21 (21): 7887.
37. Ko SF, Huang TH, Lin YP, Chen YL, Yip HK (2022) Accuracy and precision of 31P-MRS assessment for evaluating the effect of melatonin-pretreated mitochondria transferring on liver fibrosis of rats. Melatonin Res. 5 (1): 18-33.
38. Lee FY et al. (2015) Intracoronary Transfusion of circulation-derived CD34+ cells improves left ventricular function in patients with end-stage diffuse coronary artery disease unsuitable for coronary intervention. Crit. Care Med. 43 (10): 2117-2132.
39. Sung PH et al. (2018) The five-year clinical and angiographic follow-up outcomes of intracoronary transfusion of circulation-derived CD34+ cells for patients with end-stage diffuse coronary artery disease unsuitable for coronary intervention-phase i clinical trial. Crit. Care Med. 46 (5): e411-e418.
40. Yin TC et al. (2019) Extracorporeal shock wave-assisted adipose-derived fresh stromal vascular fraction restores the blood flow of critical limb ischemia in rat. Vascul. Pharmacol. 113: 57-69.
41. Ni XX et al. (2020) Protective effects of hyperbaric oxygen therapy on brain injury by regulating the phosphorylation of Drp1 through ROS/PKC pathway in heatstroke rats. Cell Mol. Neurobiol. 40 (8): 1253-1269.
42. Zhang Y et al. (2013) Hyperbaric oxygen therapy in rats attenuates ischemia-reperfusion testicular injury through blockade of oxidative stress, suppression of inflammation, and reduction of nitric oxide formation. Urology 82 (2): 489 e489-489 e415.
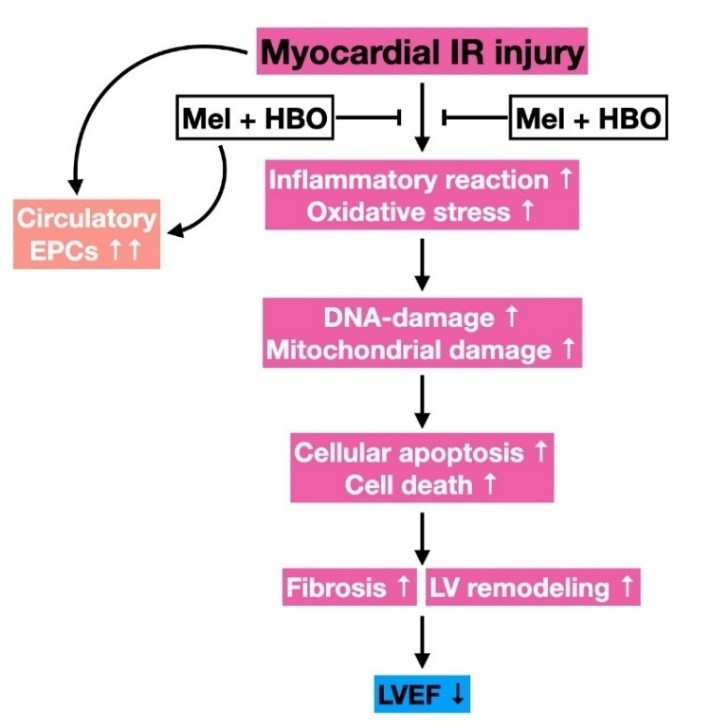

This work is licensed under a Creative Commons Attribution 4.0 International License.
For all articles published in Melatonin Res., copyright is retained by the authors. Articles are licensed under an open access Creative Commons CC BY 4.0 license, meaning that anyone may download and read the paper for free. In addition, the article may be reused and quoted provided that the original published version is cited. These conditions allow for maximum use and exposure of the work, while ensuring that the authors receive proper credit.
In exceptional circumstances articles may be licensed differently. If you have specific condition (such as one linked to funding) that does not allow this license, please mention this to the editorial office of the journal at submission. Exceptions will be granted at the discretion of the publisher.